A new method leverages an observed local supervoid to provide an additional acceleration to the universe’s expansion and address the Hubble tension.
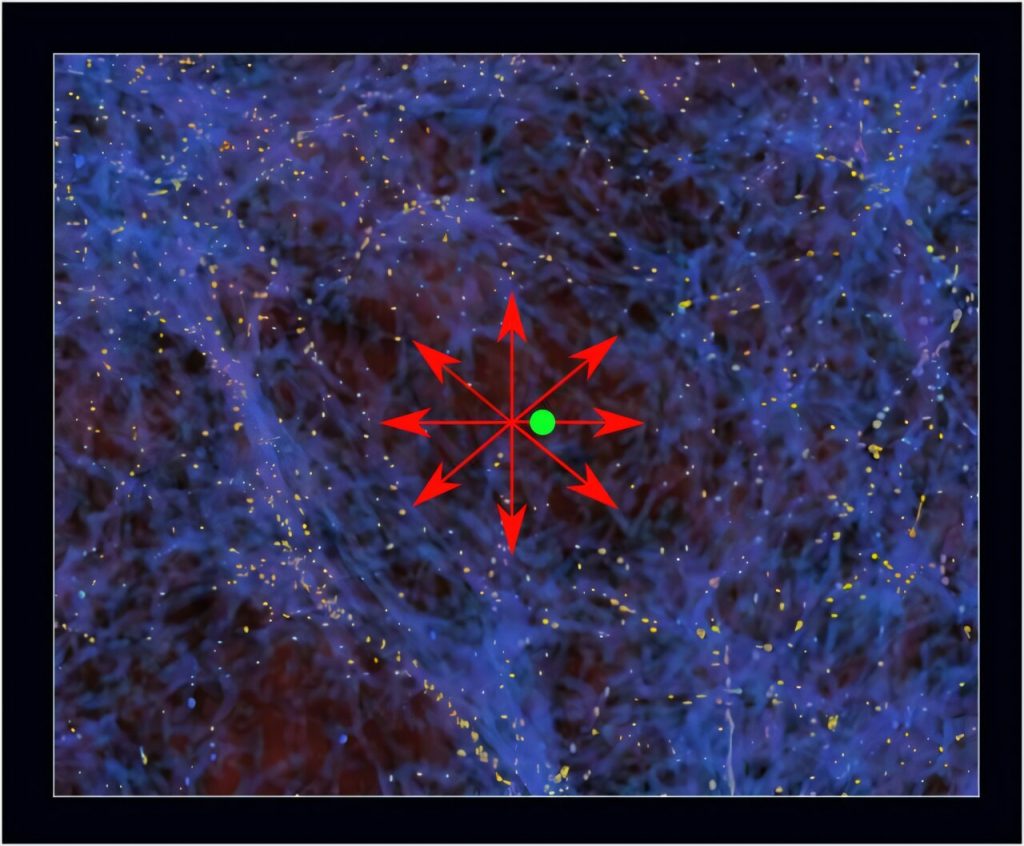
New approach uses observed local supervoid to give expansion of the universe an extra push and solve the Hubble tension (Image Credit: Phys.org)
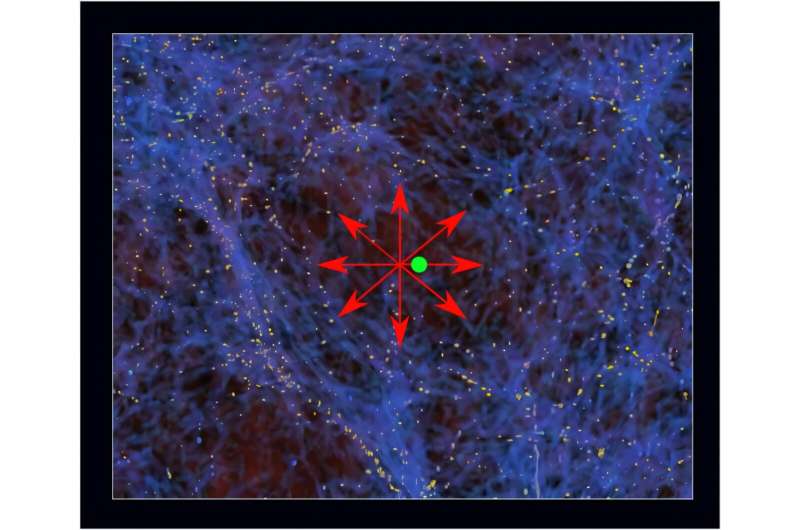
Astronomers have known for a century that the universe is expanding. We can now trace this expansion over much of its almost 14 billion year history, with important constraints going back to just one second after the Big Bang! The expansion rate today is known as the Hubble constant (H0).
We can find H0 using observations at early or late times in cosmic history, but these give different answers. This discrepancy is known as the “Hubble tension,” a real crisis for cosmology.
The early route to H0 involves the cosmic microwave background (CMB), light left over from the Big Bang. It is almost uniform whichever direction we look, but there are 0.001% fluctuations in the CMB, and these have a characteristic pattern. Just like a guitar string only makes sounds at certain frequencies based on its length, the same is true with the CMB.
By measuring which angles on the CMB sky have more “noise” and which have less, we can essentially listen to the early universe and find its size when the CMB was emitted.
Detailed modeling of this information using the standard model of cosmology known as Lambda-Cold Dark Matter (ΛCDM) shows that a good fit is possible only for a precise set of cosmological parameters, which include H0 and the densities of matter and dark energy, among other things.
This clear prediction for the present expansion rate can be tested in the nearby universe. The further away we look, the longer the light has been traveling, so the more the universe has expanded in that time.
This expansion also stretches individual photons, making more distant galaxies and supernovae look redder. By plotting this “redshift” against the distance to the supernova on a “Hubble diagram,” we get an almost linear relation known as the Hubble law. Its slope tells us H0.
This approach consistently gives H0 values about 8% higher than those based on the CMB, but the margin of error is below 2%—hence the Hubble tension.
The local measurement assumes that cosmic expansion is the only important contribution to the redshift. This does not hold for most nearby galaxies: Andromeda has a blueshift because it is approaching the Milky Way thanks to their mutual gravity, even as the universe expands on larger scales.
To minimize the effect of local structure, astronomers typically do not consider supernovae within about 300 million light years when measuring H0. But because the expansion rate has varied over time and this curves the relation between redshift and distance, they also do not go beyond 2 billion light years. This leaves a “window” in which to measure H0 from the Hubble diagram.
How a local void can help
But just how clean is this window? Perhaps not as clean as commonly believed. Observations across the whole electromagnetic spectrum strongly suggest that we are located near the center of a large region with a below average density. This cosmic void is known as the KBC void after its discoverers, who used galaxy number counts.
Matter would flow away from the KBC void towards the denser surroundings. This would create extra “peculiar” velocities on top of the cosmic expansion. These peculiar velocities would largely point away from us, creating an extra redshift that boosts the local H0 measurement.
The excess redshift would at first rise with distance, before falling away again beyond the void. We showed in a 2020 paper that this could solve the Hubble tension and explain the galaxy number counts, provided our patch of the universe is about 20% less dense than average out to a billion light years from us.
Such a large and deep supervoid is unexpected in standard theory. However, there are several hints that structures grow faster than it predicts, from giant arcs and rings of gas clouds, to galaxy cluster collisions like El Gordo that are too massive for their time.
In our models, we had to artificially jack up the strength of gravity to form the KBC void. We think this modification would only affect length scales beyond about ten million light years, where the strength of gravity is hard to test.
Discover the latest in science, tech, and space with over 100,000 subscribers who rely on Phys.org for daily insights.
Sign up for our free newsletter and get updates on breakthroughs,
innovations, and research that matter—daily or weekly.
Testing the local void model
In a recent study, we tested the local void idea further. Using our previously published local void model, we worked out the velocity field it predicts in the nearby universe. The study was published in the journal Monthly Notices of the Royal Astronomical Society.
This depends on exactly where we are located inside the void. By choosing an appropriate vantage point, we found that it is possible to gain a good match to the “bulk flow,” the average velocity of all the matter within a sphere of some fixed radius. There are some technicalities because astronomers only have access to velocities along the line of sight, but the main point is that the bulk flow can be measured without any assumption on H0.
The bulk flow depends on the size of the considered region, with results now available out to just over a billion light years. Our model provides a good fit to these observations. However, the observed bulk flow at the largest radii is quadruple the ΛCDM expectation.
We are working on several other tests. One of these uses baryon acoustic oscillations (BAOs) as a “standard ruler.” The oscillations evident in the CMB were imprinted on the distribution of matter on large scales, leading to a characteristic length scale evident in galaxy surveys. This length grows only due to the universal expansion.
By measuring the angular scale of this standard ruler at different times, we can chart the expansion history. In the local universe, a slight deviation should be apparent from a void-free cosmology because outflow from the void increases the redshift, distorting the relation between redshift and the BAO angular scale. BAO measurements over the last twenty years show just this sort of deviation.
ΛCDM cannot solve the Hubble tension or explain a void as large and deep as that observed. Perhaps cosmologists need to dig themselves out of the hole that is the Hubble tension by putting themselves at just the right spot inside a real hole.
This story is part of Science X Dialog, where researchers can report findings from their published research articles. Visit this page for information about Science X Dialog and how to participate.
More information:
Sergij Mazurenko et al, A simultaneous solution to the Hubble tension and observed bulk flow within 250 h−1 Mpc, Monthly Notices of the Royal Astronomical Society (2023). DOI: 10.1093/mnras/stad3357
Journal information:Monthly Notices of the Royal Astronomical Society
I have a Ph.D. from the University of Saint Andrews and did a postdoctorate here, as well as a Humboldt fellowship for three years in Bonn. I have worked on the possibility that we are in a billion light year radius void since 2019, motivated by observations showing the void across the whole electromagnetic spectrum and the possibility that the void solves the Hubble tension.
I now work at the Institute for Cosmology and Gravitation at the University of Portsmouth with my boss Harry Desmond on testing this idea, alongside other researchers around the world.
New approach uses observed local supervoid to give expansion of the universe an extra push and solve the Hubble tension (2024, December 1)
retrieved 1 December 2024
from https://phys.org/news/2024-11-approach-local-supervoid-expansion-universe.html
part may be reproduced without the written permission. The content is provided for information purposes only.